William Curtin, Predictive Computational Metallurgy
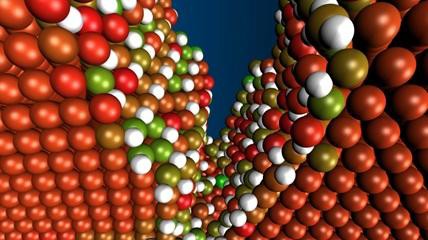
© 2013 EPFL
The excellence of the research performed at EPFL has once again been recognized at an international level. William Curtin, head of Laboratory for Multiscale Mechanics Modeling at the School of Engineering, has been awarded an ADVANCED GRANT 2013 from the European Research Council (ERC).
Predictive Computational Metallurgy
Why is there no “Moore’s Law” in the development of stronger and more durable metals? The answer is that the mechanical properties of metal alloys are almost entirely controlled by defects – dislocations, solutes, precipitates, grain boundaries, twins, and polycrystalline texture – and moreover, strength, hardening, embrittlement, fracture and fatigue are all controlled by multi-defect interactions. Designing new metal alloys requires detailed understanding of these multi-defect interactions. However, these are intrinsic core-core interactions (dislocation core, crack tip, grain boundary), occurring precisely where elasticity concepts fail, while macroscopic behaviour involves collective interactions among many such multi-defects over large length and time scales. This complexity has made metallurgy resistant to rapid progress. The PI will tackle the challenge posed by the multi-defect and multi-scale problem in metal alloys through a combined theory/multiscale simulation effort that will push forward the frontiers of computational metallurgy and yield new, quantitative, predictive models of the mechanical performance of metals alloys that will accelerate metal design. Three thrusts are proposed:
(i) Solute/Dislocation/Dislocation interactions to predict role of solute chemistry on fundamental dislocation phenomena in solute strengthened alloys, and the resulting effects on plastic flow and ductility;
(ii) Solute/Grain-boundary/Dislocation interactions to predict effects of solute chemistry on dislocation transmission/absorption and damage nucleation along grain boundaries; and,
(iii) Solute/Crack/Dislocation/Grain-boundary interactions to predict influence of solute chemistry on the propagation of cracks under monotonic and cyclic loading. The approach is to design small-scale Quantum, Atomistic, and/or Dislocation level simulations to explicitly test specific mechanistic concepts, to validate existing theories or create new predictive theories, and then create proper constitutive models. The theories and models allow for generalization of the mechanisms across materials, with required properties obtained by quantum, atomistic, and/or dislocation computations, and will enable both design of new materials and modelling of structural performance. The proposed work is feasible due to new multiscale modeling techniques developed by the PI and his recent demonstrations that this approach can yield quantitative models that explain complex long-standing problems in metallurgy.
Max ERC funding: 2.35 milion Euros
Duration: 60 months
Host institution: EPFL
Project acronym: PreCoMet
Domain: Physical Sciences and Engineering