Vladimir Manucharyan awarded Consolidator Grant
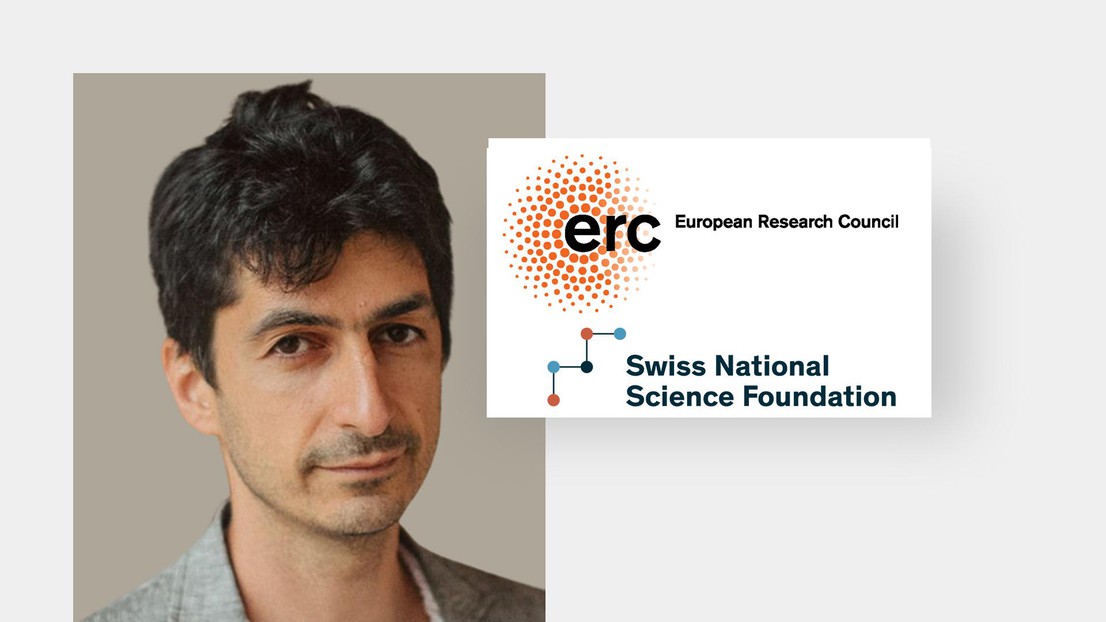
Vladimir Manucharyan. Credit: EPFL
Professor Vladimir Manucharyan at EPFL’s School of Basic Sciences has been awarded a Consolidator Grant to pursue research in Quantum electrodynamics.
The European Research Council’s (ERC) Consolidator Grants are given annually to researchers of any nationality with 7-12 years of research experience after completion of their PhD, as well as “a scientific track record showing scientific talent and an excellent research proposal”.
The Grants are part of the EU’s Horizon Europe programme, and will support mid-career researchers, helping them consolidate their teams and conduct “pioneering research on topics and with methods of their choosing.”
Due to Switzerland’s current status as a non-associated third country in the Horizon Europe research and innovation programme, the federal government has mandated the SNSF to launch the funding scheme SNSF Consolidator Grants 2023. The scheme is intended for researchers who wanted to apply for an ERC Consolidator Grant with the aim of conducting research in Switzerland and consolidating their scientific independence.
This year, one of the Consolidator Grants has been awarded to Professor Vladimir Manucharyan at EPFL’s School of Basic Sciences. Manucharyan directs the Superconductor Quantum Information Laboratory, where his research focuses on quantum computing with superconducting qubits, operating at the interface of solid-state physics, quantum optics, microwave engineering, and information science.
The grant (1,823,276 CHF) will fund a project titled “Quantum electrodynamics without photons”. The research aims to explore new physics by creating an extreme environment where photons, normally stable particles of light, no longer behave like independent particles. Using advanced superconducting technology, Manucharyan’s group plans to engineer a system where photons interact strongly, leading to complex multi-particle states.
By studying this "photonless" regime, which has never been achieved before, Manucharyan hopes to gain new insights into quantum dynamics and how particles behave in strongly interacting systems. The findings could open up new methods for studying quantum physics, without needing a fully operational quantum computer.
Some of the most intriguing phenomena in modern physics are those that cannot be described by the notion of quasiparticles, elementary excitations of the system that behave as nearly non-interacting particles. Examples usually involve strongly-interacting many-body systems in the vicinity of a quantum critical point. Extending the quasiparticle analogy to radiation, one may view photons in a non-linear media as weakly-interacting quasiparticles of electromagnetic vacuum. In fact, conventional material non-linearities are so weak that the interaction-induced lifetime of a single photon excitation (an effect known as spontaneous down-conversion) is far longer than the time of material absorption. As a result, photon has been a stable notion in all physical systems explored to this date.
What if one engineers a media with such an extreme non-linearity that would render the lifetime of a single photon shorter than all other relevant time scales in the system? In this case, the very notion of photon as an elementary excitation of the electromagnetic field becomes vague, because the field at any time is made of a potentially very complex superposition of multi-particle Fock states. The dynamics of such "photonless" electromagnetic fields is a true terra incognita in QED. It is of immense interest to quantum statistical physics in the context of thermalization in closed interacting quantum systems. Our proposal aims at implementing and exploring the aforementioned "photonless" regime of QED using recent innovations in the superconducting qubit technology: ultrahigh-impedance waveguides and highly anharmonic fluxonium qubits.
Specifically, we will engineer tunable photon-photon interaction using massively multi-mode cavity coupled to one or more qubits. When the qubit is weakly coupled, the electromagnetic modes in the cavity are well defined, while as the coupling grows and the spin-boson model approaches its dissipative critical point, the system dynamics begins to span an exponentially large many-body Fock space of multi-particle states of radiation. Such systems have not been possible previously due to the lack of non-linearity in the commonly used transmon qubits and the lack of coherence in the early experiments on the highly-anharmonic flux qubits. Our approach is based on our recent demonstration of the Altshuler-Gefen-Kamenev-Levitov non-thermalisation scenario [Altshuler et al. Physical Review Letters 78, 2803 (1997)] for a single photon excited inside an interacting multi-mode cavity [N. Mehta et al. Nature 613 (2023)].
We will pursue a broad range of spectroscopic and time-domain techniques to characterise the radiation in a massively multi-mode cavity in the regimes of weaker and stronger interactions. These include a direct spectroscopy, ancilla qubit spectroscopy, and interaction quench experiments. Using these techniques we will identify the limits on the strength of photon-photon interaction that is possible by nature (within the circuit QED framework), develop methods to tweak the connectivity of the many-body Fock space and observe the resulting thermalization and non-thermalisation scenarios. If successful, our project will introduce a new and efficient platform to study deep many-body quantum dynamics without the need for a fully controlled quantum computer. There is also a good chance of encountering new phenomena and stimulating the development of new theoretical methods in strongly-interacting QED.