Vibrational spectroscopy dissects a surface reaction
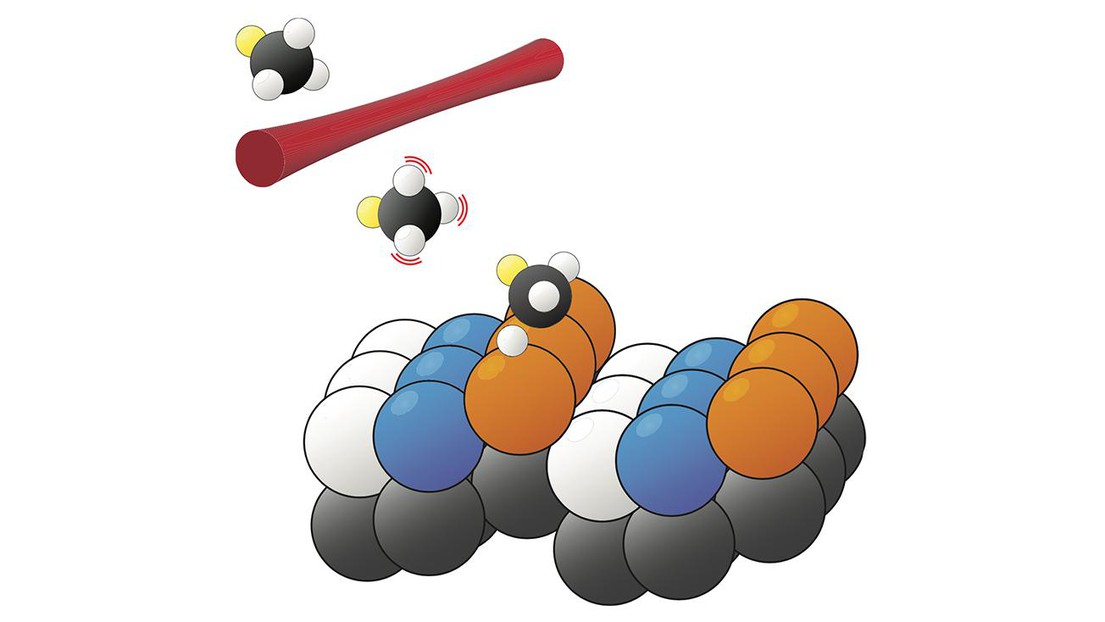
The bond-selective and surface-site-specific dissociation of deuterated methane on a Pt(211) surface (credit: R. Beck/EPFL)
When a metal surface catalyzes a chemical process, the surface atoms aren’t all the same. EPFL scientists have bridged the gap between lab and plant by studying methane dissociation on the (211) crystal face of platinum.
In 1909, Fritz Haber made a discovery that would change the world: With the help of a solid iron catalyst, he could break atmospheric nitrogen into its constituent atoms and combine them with hydrogen to make ammonia. The fertilizers produced from that synthetic ammonia increased agricultural productivity severalfold and allowed Earth’s growing population to be fed.
Industrial surface catalysis—now extended to many more reactions—is a messy process. The catalytic surfaces are rough and irregular, and hot reactant molecules jostle one another and move in all directions. Chemical physicists get a handle on the reactions by simplifying them: The craggy catalysts and pressurized reaction vessels in a chemical plant are replaced with smooth crystalline surfaces and rarefied atmospheres in the lab. The experiments have yielded some important results, including the detailed mechanism of the Haber process, but they’re a far cry from real-world conditions.
Rainer Beck, of the Swiss Federal Institute of Technology in Lausanne, and his colleagues are bridging the gap between lab and plant by studying methane dissociation on the (211) crystal face of platinum. With its regularly spaced steps, Pt(211) has three distinct types of surface sites, shown in the figure in orange, blue, and white. Previously, experiments could measure only the average reaction output of the whole surface. But earlier this year, Beck and company showed that the reaction product, CH3, had measurably different vibrational resonances depending on the surface site where it was located. By monitoring the vibrational spectrum of the whole surface and resolving it into site-specific components, the researchers probed the catalytic activity of each type of site. Qualitatively, the results fit with expectations: The step sites (orange) are more active than the terrace sites (blue), and the corner sites (white) don’t contribute to the reaction at all.
Now Beck and colleagues have added a new layer of complexity. By replacing CH4 with deuterated methane, CH3D, they have created a system in which more than one dissociation reaction is possible: The bond that breaks could be either C–H or C–D. The ability to steer such a reaction toward a desired set of products is a long-standing goal. In the case of deuterated methane, one can create a preference for C–H bond dissociation by preparing the reactant molecules in a state in which the C–H bonds vibrate strongly but the C–D bond does not. But the degree of that preference exhibits a complicated dependence—which Beck and colleagues have now disentangled—on both the reaction site and the reactant speed.
The Lausanne experimenters are working with several theory groups to convert their results into a better predictive understanding of surface reactions. And they’re extending their experiments to even more complicated surfaces, such as Pt(531), whose steps are zigzag-shaped.
Reproduced from J. L. Miller, ‘Vibrational spectroscopy dissects a surface reaction,’ Physics Today Online, 26 July 2018, with the permission of the American Institute of Physics. [https://doi.org/10.1063/PT.6.1.20180726a].
Fonds national suisse de la recherche scientifique (FNS)
École Polytechnique Fédérale de Lausanne (EPFL)
Ana Gutiérrez-González, F. Fleming Crim, Rainer D. Beck. Bond selective dissociation of methane (CH3D) on the steps and terraces of Pt(211). Journal of Chemical Physics. In press.