Vanishing worlds: Exploring our planet's icy ecosystems
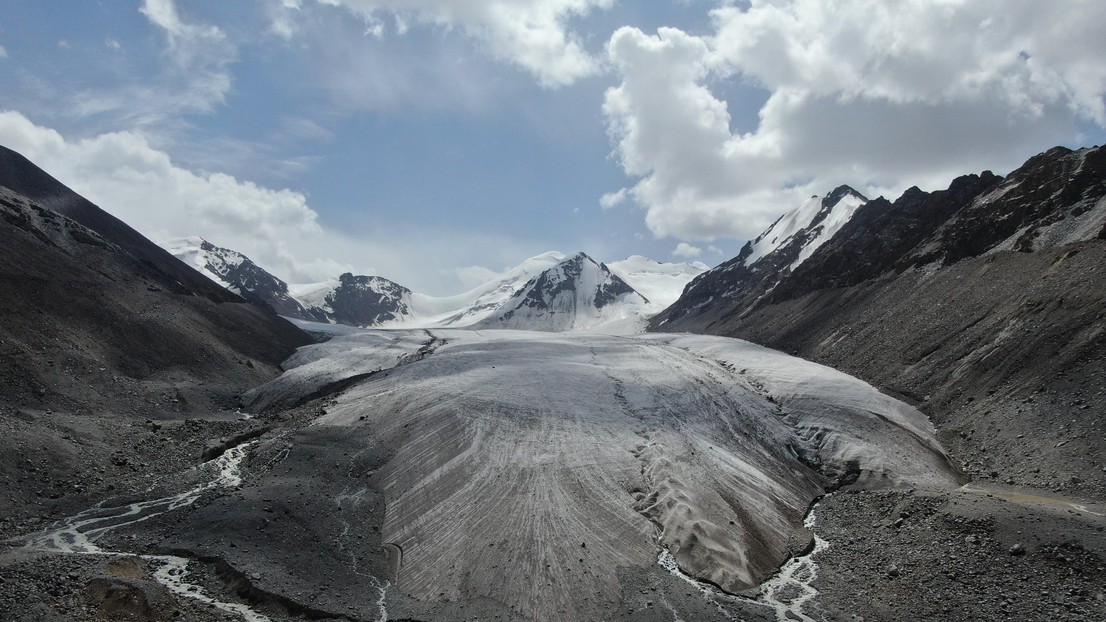
Kyrgizstan, Arabel Plateau © Vincent de Staercke - EPFL
Long considered devoid of life, glaciers – and the microorganisms they harbor – are receiving the recognition they deserve.
It all begins with a speck. Brought in by the wind, dust, soot, or other particulate matter deposits on the ice. The newly arrived nutrients awaken microorganisms – bacteria, algae or fungi – that lie dormant on the surface. As their metabolisms produce organic matter, the speck grows, absorbing more sunlight and burrowing deeper into the melting ice. The dimples formed fill with nutrient-rich meltwater, fueling further growth. The result is an ecosystem-in-a-puddle, or cryoconite – a fascinating demonstration of glacier landscapes shaping and being shaped by their microscopic inhabitants.
Ice covers one tenth of our planet’s surface. Still, says Tom Battin, who leads EPFL’s River Ecosystems Laboratory, we’ve only just begun to catalog the myriad species these frozen habitats are home to. “Glaciers are our water towers, and the streams they feed are the taps that deliver fresh water to hundreds of millions of people. But they are more than just that, and it’s time we understand what else we will lose when the ice melts. What species live up there, and what biodiversity is at risk? For that, we need to conduct a thorough census of the local biodiversity.”
Teeming with life, above, within, and below the ice
Until a few decades ago, glaciers were often absent in ecology textbooks. Today, we know they are teeming with microbial life. At their surface, bacteria, algae, and fungi take full advantage of the short growing season. Sometimes visible to the naked eye as cryoconites or red-colored snow, they mobilize a rich repertoire of tools to harvest solar energy. Down below, the glaciers’ relentless flow crushes the underlying bedrock in “mineral factories” replete with nutrients that serve as a source of energy for specialized bacteria. And within the ice, often several kilometers deep, microbes form pockets of life within three-dimensional networks filled with circulating liquid brine.
For just over two decades, Battin has been studying these microbial glacier ecosystems. He recently led the first systematic global census of microbial diversity in glacier-fed streams that took him and a network of researchers to all four corners of the globe. “With support from the Nomis Foundation, we were able to sample glaciers in the Americas, Europe, Asia, New Zealand and Africa. By combining cutting-edge molecular biology methods with phylogeny, we assembled a unique dataset that lets us identify global patterns and, for the first time, get a comprehensive view of these threatened ecosystems. It’s a game changer,” he says.
By studying the microorganisms that make up glacier ecosystems, researchers hope to better understand the strategies they have evolved to scavenge energy in some of the planet’s harshest environments, overcoming sub-zero temperatures, scarce organic nutrients, ultraviolet radiation and frequent freeze-thaw cycles. “The species we find in glaciers often have a very plastic metabolism that lets them switch from sunlight-harvesting photoautotrophs to organic carbon-consuming heterotrophs. The chemolithoautotrophs, meanwhile, survive by drawing energy from inorganic compounds.”
By combining cutting-edge molecular biology methods with phylogeny, we assembled a unique dataset that lets us identify global patterns and, for the first time, get a comprehensive view of these threatened ecosystems. It’s a game changer.
Ecosystems at risk: Implications of melting ice
By transforming inorganic compounds – carbon, nitrogen and sulfur – into more readily available organic ones, these microorganisms provide vital nutrients for food webs of growing complexity further downstream. Just how much is at stake as their habitats disappear is not yet fully understood. But, says Battin, “the bottom line is that as glaciers melt, this vast biodiversity is being homogenized as specialized microbes, algae and invertebrates they harbor are replaced by generalists that can move upstream and colonize these newly ice-free habitats.”
Their disappearance also comes with a loss of hitherto unrecognized genetic potential that could benefit humanity. “Biotechnology firms are interested in bioprospecting these bacteria, for example, for enzymes capable of catalyzing chemical processes at low temperatures. These could increase the energy efficiency of industrial processes by lowering the required processing temperatures. Similarly, pharmaceutical companies hope to find new antibiotics in these remote and pristine environments.”
Overcoming challenges on Earth and beyond
That’s one reason why ongoing initiatives seek to preserve these microorganisms in biobanks, says Ianina Altshuler, Director of EPFL's Laboratory of Microbiome Adaptation to the Changing Environment (MACE), who studies permafrost ecosystems. The task is challenging: “We are only able to culture and preserve a small percentage of microbial species, around one to two percent” she says. Taking stock of active microorganisms in their cryospheric niches is no less complicated. “It’s a big challenge to identify the organisms that are active in these ecosystems, for example, within brine veins in the permafrost, because there are so few of them relative to the organisms that are dead or dormant in the substrate surrounding them.”
The experience gained studying these microbial ecosystems in their harsh habitats could one day prove valuable to research carried out much, much farther afield. “Cryospheric environments on Earth are analogue sites that we can use to test devices that could be payloads on missions searching for life on Mars or Enceladus, Saturn’s icy ocean moon,” says Altshuler. Working with a team of researchers, she participated in developing a semiautomated life-detection system capable of collecting and processing environmental samples and detecting the fingerprints of biological life. Designed such that it can be incorporated into a moon rover, the instrument demonstrated its capabilities in the Canadian high Arctic.
Training the next generation of cryosphere scientists
In the meantime, the researchers will continue to study icy ecosystems right here on Earth. “The goal of the ongoing ICEBIO project, carried out with partners in Denmark, Germany, France, Austria and the UK, is to train a generation of young scientists that is aware that ice is not just frozen water, but also home to a complex biome. By studying small-scale feedback loops and investigating their big-picture implications, participants will try to predict how these ecosystems could change as warming continues,” says Battin, who is supervising two PhD students funded by the ICEBIO Marie Skłodowska-Curie Doctoral Network.
Their work has involved fieldwork in Tajikistan as part the Swiss Polar Institute’s PAMIR Tajikistan expedition and, closer to home, coring ice from five glaciers in the canton of Valais and sampling the streams they feed to compare the species detected. It’s a tedious process, alternating between days of field measurements followed by months of downstream work, extracting and sequencing DNA, and analyzing the data using bioinformatic tools. Meanwhile, a second project focuses on exploring stream habitats to better understand golden algae, a “forest-forming” unicellular organism whose unique biophysics makes it particularly adapted to the turbulent flows it thrives in and that is heavily colonized by bacteria.
A clearer picture of the microbiological processes that unfold in the cryosphere could offer insights into the greening of post-glacial ecosystems increasingly observed by ecologists. As glaciers shrink, they mobilize less minerals, allowing a greater fraction of incoming sunlight to shine through the less turbid water, making the riverbed a more hospitable environment for primary producers, effectively establishing “green oases” as photosynthesizing photoautotrophs replace mineral-mining chemolithoautotrophs. It’s a rare transition from a grey to green ecosystem that environmental researchers are reluctant to applaud.