Uncovering the origins of friction
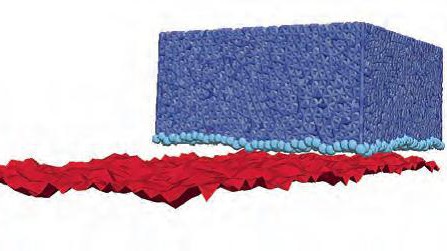
© 2013 EPFL
Jean-Francois Molinari is taking friction from a recipe-driven measurement to a force whose physics is incorporated on a much more fundamental level.
“Wherever you have contact, you have friction and wear,” announces Jean-Francois Molinari, a young French-born professor who joined EPFL’s ENAC school faculty in 2007. Friction is an expression of the forces acting when subatomic particles rub shoulders. Anything that touches anything else is subject to this ubiquitous force – roads, joints in structures, railroad tracks, waves crashing on a beach. The flame sparked when you rub two sticks together, the relentless scouring of a mountainside by a glacier, the devastating damage of earthquakes and tsunamis – all caused by friction. “Maybe because it’s everywhere, we take it for granted,” he shrugs. “But it’s very, very important. Probably 5% of GDP is wasted by replacing components that degrade via friction.”
Despite the fact that it affects everyone and everything on the planet (and beyond), friction is still not very well understood. “It’s one of the greatest unsolved mysteries in science,” Molinari insists. Why is friction such a sneaky force? To begin with, it operates at multiple time and length scales. In order to model it, you have to know exactly how much of a surface is touching another surface. Take a book sitting on a desk, for example. Believe it or not, only about 1% of the book’s surface is actually in contact with the desk. This is because the two surfaces are very rough. Fractal models are the perfect way to describe rough surfaces, all the way down to the atomic scale. But elegant as this solution may appear, it’s not at all practical. Fractals are like mirrors inside mirrors – as you endlessly go deeper and deeper, smaller and smaller, the computer time starts to pile up, the centuries go by, and you never actually reach the end. Even if those surfaces could be modeled perfectly, Molinari says, we’d still have trouble, because the friction force itself isn’t just a simple calculation. And there are many different kinds of friction, depending on the type of surface involved. “It’s extremely complex physics,” he admits. “Different dissipative mechanisms are taking place, resulting in lots of different physical phenomena.” The traditional way to get a grip on friction is to run experiments and measure the resulting “friction coefficient.” Although this quantity helps all the equations balance out, it doesn’t reveal much about the actual physics taking place. “But there’s hope,” he smiles encouragingly. Our increasing obsession with nanotechnology has led to new techniques, such as atomic force microscopy, that allow us to measure frictional forces at tinier and tinier scales. In 2008, scientists managed to move a single atom across a surface and measure the forces involved. Thanks to these improvements, numerical simulations have progressed considerably.
Molinari’s research is at the vanguard of these simulation efforts. He’s taking friction from a recipe-driven measurement to a force whose physics is incorporated on a much more fundamental level. Using high performance computing to run parallel scalable algorithms, he is combining molecular dynamics at the very small scales with continuum mechanics at the larger scales. The result: the first physics-based, parallel, multiscale simulation platform for directly computing the friction force. It is incorporated into an open software hub, so that it can be used for a number of practical applications – to design surfaces with specific adhesive properties, to optimize surface finishes, to design biological lubricants and materials, for example. Molinari also hopes it can be used to shed light on problems that cannot be studied experimentally, such as our inability to predict temperature increases in earthquakes.