Thale cress: The unassuming weed that's lighting up science
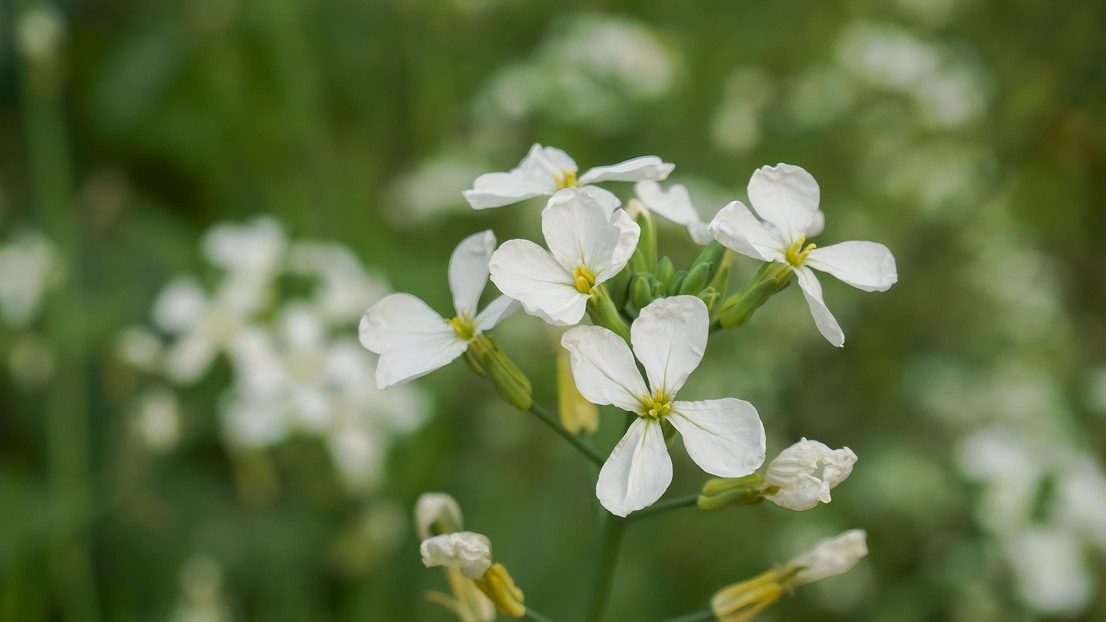
© 2024 EPFL
It’s a well-established fact that, in the plant world, competition for sunlight is intense. Smaller plants grow taller and spread their leaves to escape the shade cast by their loftier counterparts. But while we’ve long understood the mechanics of this adaptation process, little was known about how plants detect where sunlight is coming from. A team of scientists found the answer deep inside the cells of Arabidopsis thaliana.
Read the enhanced version of this article
In a groundbreaking collaborative research project, UNIL biologists and EPFL engineers provided the first-ever demonstration of the correlation between light-scattering patterns and plants’ ability to turn towards the sun. In doing so, they answered a fundamental question: how do plants know where to find light? Their findings were published in the journal Science.
The EPFL team was led by Andreas Schüler, a senior scientist who heads the Nanotechnology for Solar Energy Conversion research group. Working with biologists led by Prof. Christian Fankhauser, a plant development expert at UNIL’s Center for Integrative Genomics, they spent several months conducting interdisciplinary research in an effort to solve this age-old mystery.
“We knew that the thale cress stem was made up of cells arranged around tubes of air,” says Prof. Fankhauser. “What we didn’t know was how the light scatters inside the plant, or how it transmits information to the cells to activate different responses so the plant can orient itself toward the strongest light source.”
Although phototropism – the ability of plants to turn towards light – is a well-known concept, the process by which the photoreceptors on either side of the plant are activated has always been poorly understood. “The cells that respond to light in plants are called phototropins,” explains Martina Legris, a PhD student at UNIL. “Phytochromes are receptors that respond to red light, while cryptochromes are sensitive to light at the blue and UV-A end of the spectrum – the part that influences plants’ circadian rhythm. Phototropins also play a role in leaf positioning and provide information about the brightness of the light.” However, these established facts didn’t tell scientists enough about what was actually happening inside the cells.
As is often the case in science, the breakthrough came by chance. The UNIL team happened to create a mutant thale cress strain that was waterlogged and translucent. The observation that this variant was incapable of turning towards a light source provided the starting point for their research. But the team hit a stumbling block when it came to calculating variations in brightness – known as the light gradient – at different locations in the plant’s tiny, fragile stem. “We tried several options, such as using pigments and working with the plant’s optical properties, but to no avail,” recalls Prof. Fankhauser. “That’s when we decided to turn to Andreas Schüler for help. His team had the instruments we needed to measure these optical properties much more precisely.”
Testing the plant like a solar collector
Fankhauser and Schüler had previously met at a conference on natural light – a shared research interest that served as a springboard for this new project. “It’s always exciting to do this kind of interdisciplinary research,” says Schüler. “It took us outside our comfort zones.” For engineers accustomed to working with solar collectors, analyzing plant-stem sections less than 1 centimeter in length and just 250 microns across was no easy task. The EPFL team had to reconfigure some machines and learn how to fix the samples without damaging or altering them. But, as Schüler explains, the overall approach was the same: “In our work on solar collectors, we study interactions between light and structured materials at the micro and nano scales. Likewise, biologists are interested in how light interacts with different parts of plants.”
The team’s first step was to take CT scans of the tiny mutant thale cress and its wild relative. This allowed them to build a detailed picture of their respective structures without destroying the samples. Next, they subjected the plant to a battery of measurements, including placing the sample inside an integrating sphere – an optical device, also known as an Ulbricht sphere, that’s used in photometry to measure surface luminance. This instrument tells researchers how bright a given surface appears when viewed from a particular angle, taking into account factors such as how much light is emitted or reflected by the surface, and how this light is distributed in space. The device works by gathering light from a source, which then bounces off the sphere’s white inner walls to produce multiple scattering reflections.
Plants are smart
“We combined this approach with our spectrometer to break up the light beam into its constituent wavelengths,” explains Schüler. “The sample was so small and the amount of light was so low that we had to find a more powerful source. Nevertheless, we successfully demonstrated a correlation between light-scattering patterns in the micrometer-scale air tubes and the plant’s ability to move toward the light source.”
“Our model shows that the air tubes play a key role in enabling the plant to precisely perceive a light gradient and sense its direction. This mechanism wasn’t previously known to science,” says Fankhauser. When saturated with water, the plant loses its phototropic ability because the light is absorbed and scattered. For Prof. Fankhauser, this discovery is yet another example of plants’ limitless capacity for adaptation and survival: “Plants are smart. They can also adjust their direction of growth by sensing gravity, in a process known as gravitropism. That’s why, if you take a stem and lay it down flat in a dark room, with no light information, it’ll still end up growing vertically.”