Neurons warp space and time
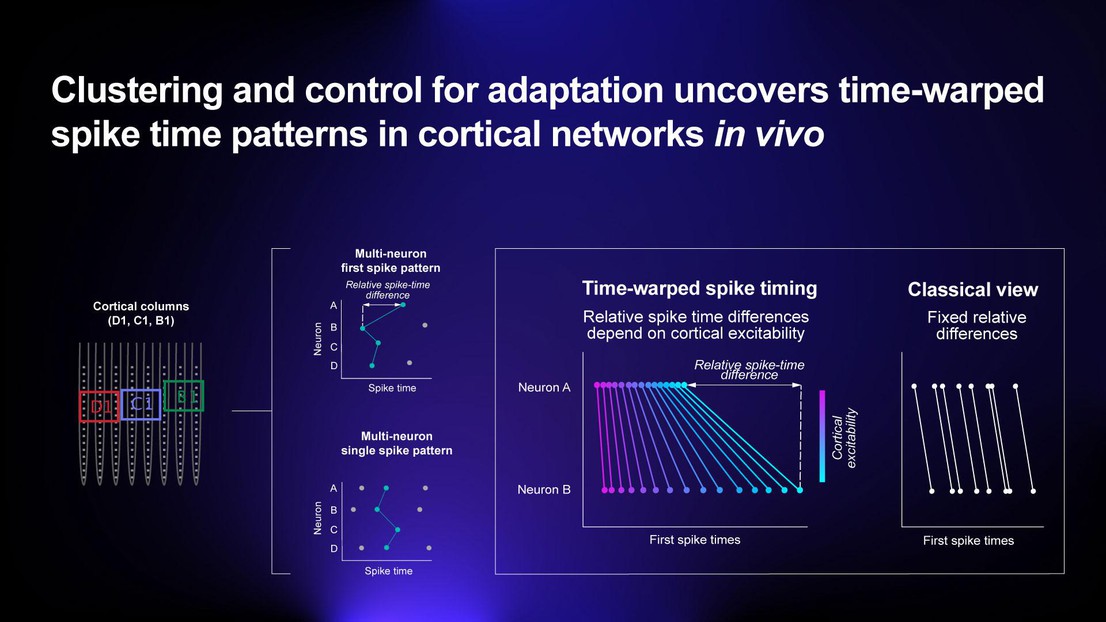
© 2022 EPFL
The messages sent between neurons look something like a Morse code, but the closer one looks the more mysterious the code becomes. The code is called the neural code and is the holy grail in neuroscience because if this code is cracked, then one could simply intercept the messages and
decode what the brain is seeing, feeling, and thinking. Theories have fallen from grace one after another for over a century. But now researchers may have uncovered that the mystery may come from the way that time is used to represent information in the brain.
In Morse code, a single `sender’ encodes a message in a long sequence of `dots’ and ‘dashes’. A single paragraph of text may correspond with sequences of Morse code lasting minutes. Neurons, on the other hand, must rapidly convey information and make decisions. To encode information quickly, neurons work together in groups to produce sequences of activity lasting 20-50 milliseconds. Within such sequences, each activated neuron contributes only one or several “dots” to the sequence. In neuroscience, these “dots" are known as “spikes”.
How then is information encoded by such sequences of spikes? Classically, many researchers focused on the number of spikes that each neuron emitted. A smaller group of neuroscientists instead endeavored to explore whether information was encoded by the precise timings of individual spikes within a sequence. Often, however, the timings of spikes within a sequence were unreliable and appeared to be more random than expected, leaving open the question of how information might be more intricately encoded by the timings of spikes.
In a paper published in Nature Scientific Reports, a group of scientists from the EPFL Blue Brain Project, University of Oxford, Johannes Gutenberg University, Universita della Svizzera Italiana, and NERF/IMEC, Belgium, have shown that the timings of spikes are much less random than was previously thought. They discovered (in vivo) that the times that individual neurons spiked within a sequence depended on the excitability of the cortex when a stimulus was presented.
When the cortex was more excited, spike sequences were compressed: evolving quickly in time. When the cortex was less excited, the spike sequence was stretched: evolving slowly in time. Moreover, as the spike times of different neurons were affected by this stretching and compression by different amounts, the order of spikes also depended on the excitability of the cortex. The authors refer to this compression and stretching of spike sequences in time as “time-warping”. Time-warping may explain why reliable and precise spike sequences have rarely been reported.
To form a full theory of neural coding, the authors considered how “receiving” neurons might interpret a time-warped spike sequence. The finding that spike times of neurons separated over layers and columns of cortex are modulated by the same changes in excitability, introduced the possibility that neurons, which receive a sequence of spikes, are also modulated by the same changes in excitability. These receiving neurons would then have knowledge of the degree of compression or stretching that the incoming spike sequence had undergone, and could potentially use this knowledge to better understand what information the group of sending neurons was trying to convey.
Finally, the authors highlight that most neurons contribute only one spike to a sequence when a short sensory sequence is presented. Such time-warped sequences (in which each neuron contributes a single spike) and which are interpreted depending on the current excitability-level of the cortex, may be a fundamental form of information coding in the brain. The novel proposal of “time-warped multi-neuron single spike patterns" as a fundamental form of cortical information representation introduces a new theory of neural coding, which accounts for a wide body of historical results and may explain one of the ancient mysteries of neuroscience.
In vivo toin silico
Lead researcher, James Isbister, joined Blue Brain in 2020 and is now aiming to use in silico cortical models to explore the cortical representation of sensory information and to make predictions about in vivo neural activity. “I am currently developing tools to compare in vivo and in silico cortical models, which will act as a validation tool for in silico cortical models”, he reveals. “Furthermore, these tools will also guide data-driven refinement and development of in silico cortical models. At Blue Brain we are excited to explore how in vivo and in silico spiking activity can inform one another and help elucidate the rules by which information is encoded in the brain.”
“For the Blue Brain Project”, explains Blue Brain Founder and Director Prof. Henry Markram, “An accurate in silico cortical model would capture the fine temporal structure of the in vivo neural activity. If the model could recreate this fine temporal structure, this would provide a very strong validation of the model and could be used to explore the nature of such representations beyond what is possible with in vivo experiments. The model could also be used to make predictions, including about the representations underlying mechanisms and purpose, with in vivo and in silico complementing and informing one another. The findings and potential future research directions are exciting,” he concludes.
____________
Citation: Isbister, J. B., Reyes-Puerta, V., Sun, J.-J., Horenko, I., & Luhmann, H. J. (2021). Clustering and control for adaptation uncovers time-warped spike time patterns in cortical networks in vivo. Scientific Reports, 11 (1), 15066. https://doi.org/10.1038/s41598-021-94002-0
James B Isbister’s contribution to this work was supported by the Oxford Foundation for Theoretical Neuroscience and Artificial Intelligence, the UK Economic and Social Science Research Council (ESRC) (Grant No. ES/J500112/1) and the UK Engineering and Physical Science Research Council (EPSRC) (Grant No. EP/N509711/1), and funding provided by the Swiss government’s ETH Board of the Swiss Federal Institutes of Technology to the Blue Brain Project, a research center of the École polytechnique fédérale de Lausanne (EPFL). Illia Horenko and Heiko J. Luhmann are supported by Deutsche Forschungsgemeinschaft and Carl-Zeiss grant EAI to the University of Mainz.