Locating precise catalytic sites in steam reforming
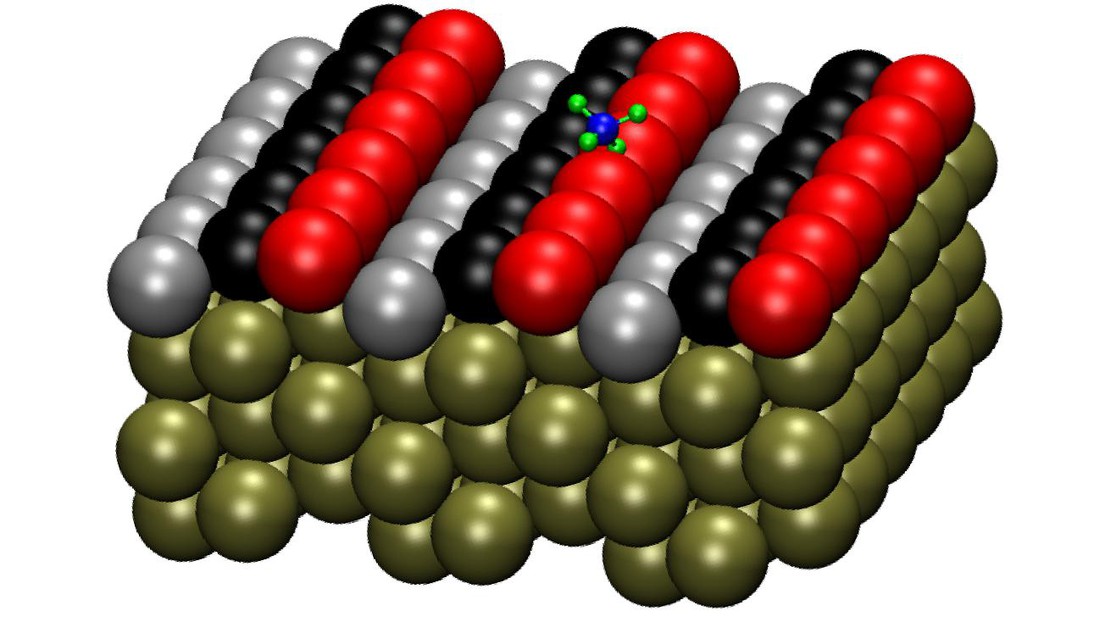
The Pt(211) surface model with the methane molecule on it ©Rainer Beck/EPFL
Using infrared spectroscopy and a quantum theory model, EPFL scientists have gained a more complete understanding of methane dissociation reactions on platinum. The work is published in The Journal of Chemical Physics.
Transition metals, such as nickel and cobalt, are known for their superior chemical bonding capabilities. Because of this, these are widely used in the industrial production of hydrogen and other useful compounds from natural gas. The most common method for achieving this transformation is "steam reforming": methane, which is the main constituent of natural gas, is heated with steam in the presence of the transition-metal catalyst, and is converted into hydrogen and carbon monoxide.
There is always the search for better transition-metal catalysts, and the search is largely based on trial and error. Scientists know that the most significant reactions occur at the surface of the catalysts, but much of the guesswork is based on the assumption that catalyzed reactions take place on atomic defect sites of the metal crystals.
The lab of Rainer Beck at EPFL, with colleagues at the University of Massachusetts, combined infrared techniques with quantum theory to explore these reactions in unprecedented detail. Their work reveals for the first time exactly where the most significant reactions occur on the catalyst’s surface.
The researchers focused on platinum (Pt) as the catalyst for breaking down methane. At the atomic scale, the surface of a platinum catalyst, as well as other metal crystals, can consist of various defects such as steps and terraces, all of which are thought to be important “sites” in the catalytic process.
Using infrared laser pumping, the researchers excited methane molecules into selected rotational and vibrational quantum states. They then detected methane dissociation on the various sites of the Pt(211) crystal by using reflection-absorption infrared spectroscopy (RAIRS), a non-intrusive technique that allows researchers to monitor chemical reactions in real time during the deposition of, in this case, methane on the platinum's surface. This is done by recording site-specific uptake curves for chemisorbed methyl species on steps and terraces sites, which can then be used to determine the reactivity levels of methane on each of the sites.
“We demonstrated that it is possible to use RAIRS detection for state- and surface-site specific measurements of methane reactivity and to compare the effect of vibrational excitation on reactivity on the steps and terraces of a catalyst surface,” says Rainer Beck. “This new area of study provides another level of detail in detecting methane’s dissociation products.”
The researchers combined RAIRS with a quantum theory framework (Reaction Path Hamiltonian model) to calculate the potential energy surface and explore the dynamics during the chemical reactions. The approach showed that dissociation reactions are at least two orders of magnitude more efficient on the atomic steps than on the terraces. A third type of surface site located between steps and the terrace, referred to as “corner atoms”, saw no reactions taking place.
Although the study looked at platinum, the model can be applied to other transition-metal catalysts, such as nickel. “A tested predictive theory with chemical accuracy could change the way one searches for new catalysts and make the search more efficient and cheaper,” says Beck.
Funding
- Swiss National Science Foundation (FNS)
- Ecole Polytechnique Fédérale de Lausanne (EPFL)
Reference
Helen Chadwick, Han Guo, Ana Gutiérrez-González, Jan Paul Menzel, Bret Jackson, Rainer D. Beck. Methane dissociation on the steps and terraces of Pt(211) resolved by quantum state and impact site. The Journal of Chemical Physics 148, 014701 (2018). DOI: 10.1063/1.5008567