Imaging a killer: High-resolution structural analysis of Huntingtin
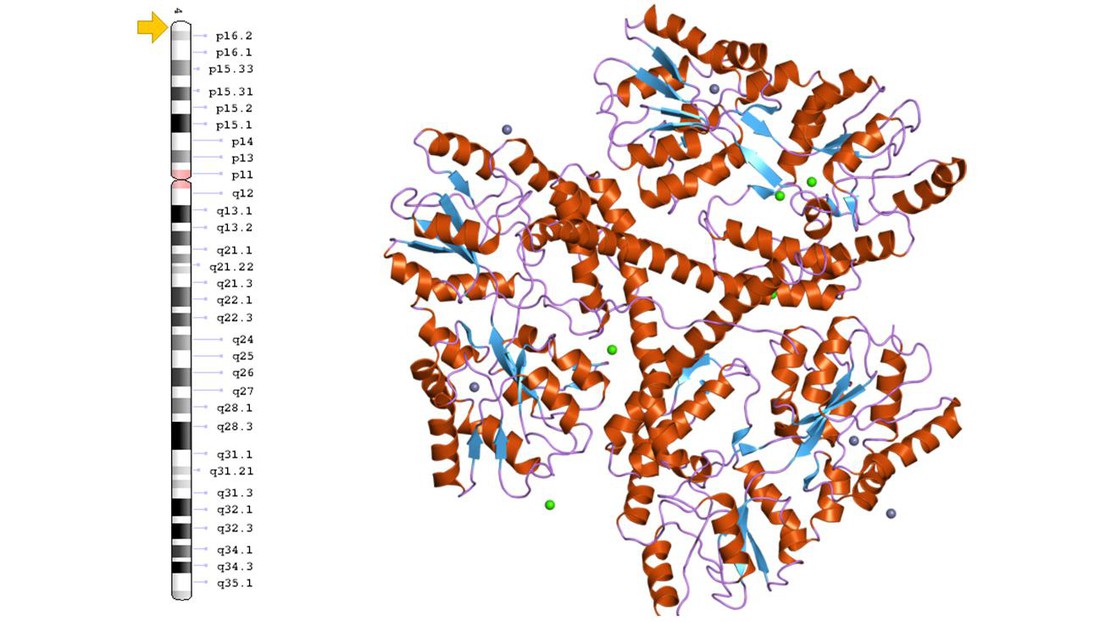
The location of the htt gene on chromosome 4 (credit: Genetics Home Reference/NCBI) and the 3D structure of huntingtin (EBI)
An international team of researchers has obtained the first ever atom-level structural insights into bona fide monomeric forms of Httex1, a part of the huntingtin gene that is thought to underlie Huntington’s disease.
Huntington’s disease is a progressive, fatal neurodegenerative disorder that is caused by mutations in one specific gene called huntingtin (Htt). In the 20-plus years since the Htt gene was identified, researchers have focused on the protein encoded by the Htt gene, called Httex1. This protein accumulates in the brains of Huntington’s disease patients, and the prevailing hypothesis has been that it undergoes a dramatic structural change when a repetitive tract of the amino acid glutamine mutates into an aberrantly long region known as the mutationally expanded— polyglutamine (polyQ) tract.
Now, for the first time, the team of lab of Hilal A. Lashuel at EPFL, Edward A. Lemke at the European Molecular Biology Laboratory (EMBL) in Germany and Rohit V. Pappu at Washington University in St. Louis, has uncovered a detailed structural description of Htt as a function of polyQ length. The work was published recently in the Journal of the American Chemical Society.
A study in three steps
Obtaining atomic-level structural descriptions of full-length Htt or disease relevant protein fragments referred to as Httex1 has been challenging because these molecules stick to one another and inhibit the generation of pure protein samples for structural studies. “It is very difficult to obtain structural characterization of proteins within a mush,” says Rohit Pappu, the Edwin H. Murty Professsor of Engineering at Washington University in St. Louis.
“Our goal was to gain insight into the how increasing the length of the polyQ tail repeat alters structure of this protein at the monomer level and under conditions where we are able to unlink its folding and self-assembly,” says Hilal Lashuel, Professor of Life Sciences and Director of the laboratory of the chemical biology of neurodegeneration at EPFL.
In the first step of the study, Lashuel and his postdoctoral fellow John B. Warner IV used novel chemical strategies to produce precise, high-purity samples of Htt for molecular spectroscopy. But these only came in ultra-low concentrations, and required techniques that probe individual molecules. Warner and Lashuel enabled these experiments by generating samples with site-specific fluorescent labels.
For the second step of the project, Warner and Lashuel worked with the group of Edward A. Lemke at EMBL to perform single-molecule Förster (or fluorescence) resonance energy transfer (smFRET), which is a technique that can measure distances between 1-10 nanometers within individual molecules – in this case, within individual Htt proteins. This part of the study yielded the first quantitative assessment of how the inter-atomic distances within Httex1 vary with the expansion mutations.
Finally, the scientists worked with Pappu’s lab at Washington University in St Louis, who developed novel computer modeling approaches to produce physically accurate, atomic-level structural models of Httex1 that best fit all of the single-molecule data from the previous two steps. The results were surprising: The overall structure of Httex1 resembles that of a tadpole.
The tadpole effect
“Architecturally, Httex1 is tadpole-shaped, with a globular polyQ head and a floppy tail,” says Pappu. “As the polyQ length gets longer the head of tadpole becomes larger in surface area. This increased surface area of the head appears to engender interactions that otherwise shouldn’t be present in cells.”
The discovery challenges the longstanding ideas about Httex1 accumulation in Huntington’s disease. “If the prevailing hypothesis were true,” explains Pappu, “then the tadpole would have turned into a ‘frog’ as the polyQ length increases above the threshold length, but that does not appear to be the case. The new results instead focus our attention on the novel gain-of-function cellular interactions that are driven by the tadpole structure with a larger polyQ head.”
“While the prevailing hypothesis has favored a model where mutant huntingtin induced toxicity is driven mainly by its propensity to misfold and aggregate, our findings suggest that aberrant interactions at the monomer level may also contribute to the initiation and/or progression of the disease”, says Lashuel.
“This finding allows us to examine what regions of this protein are important to target, and modulate its toxicity in a specific manner,” says Kiersten M. Ruff, the postdoctoral fellow in Pappu’s lab who designed the computer simulations and is the co-first author on the paper.
The next challenge for the scientists is to understand how these structural changes at the monomer level of Httex1 translate into increased aggregation and toxicity when the length of the polyQ tail crosses the pathogenic threshold.
“The key has been the centrality of collaboration among three teams with complementary and non-overlapping expertise a shared commitment to advancing science,” says Lashuel.
Contributors:
- École Polytechnique Fédérale De Lausanne (EPFL)
- Washington University in St. Louis (WUSTL)
- European Molecular Biology Laboratory (EMBL)
Funding
- CHDI
- EPFL
- US National Institutes of Health
- EMBL (EIPOD, under Marie Curie Actions COFUND)
Reference
John B Warner IV*, Kiersten M. Ruff*, Piau Siong Tan, Edward A. Lemke, Rohit V. Pappu§, Hilal A. Lashuel§.(*Co-first authors; §Co-corresponding authors) Monomeric huntingtin exon 1 has similar overall structural features for wild type and pathological polyglutamine lengths. Journal of the American Chemical Society 22 September 2017. DOI: 10.1021/jacs.7b06659