Gaining insight into the energy balance of earthquakes
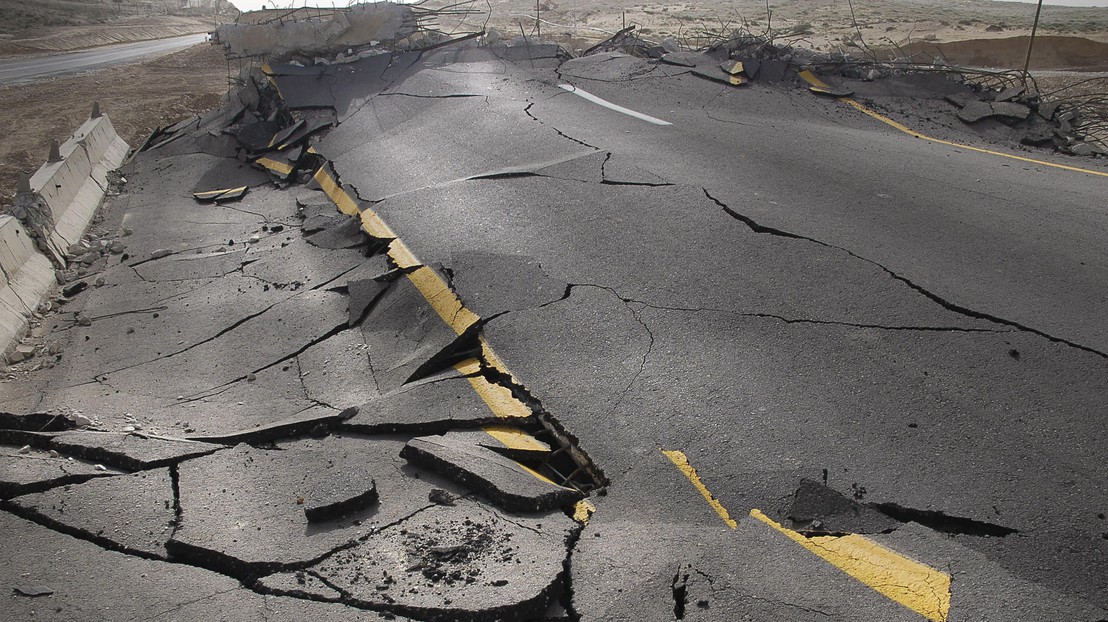
© 2019 iStock
Researchers at EPFL’s Computational Solid Mechanics Laboratory and the Weizmann Institute of Science have modeled the onset of slip between two bodies in frictional contact. Their work, a major step forward in the study of frictional rupture, could give us a better understanding of earthquakes – including how far and fast they travel.
It’s still impossible to determine where and when an earthquake will occur. For example, California has for years been under the threat of the “Big One,” and closer to home, a recent series of small shocks in Valais Canton in early November has raised fears of a major earthquake in the region. Although we can’t predict earthquakes, researchers from EPFL and the Weizmann Institute of Science in Israel have made a step forward in assessing earthquake dynamics through a better understanding of how frictional slip – the relative motion of two bodies in contact under shear stress, such as tectonic plates – begins. Their work has been published in two complementary parts, in Physical Review X and Earth and Planetary Science Letters.
“We wanted to understand what happens when two bodies in frictional contact suddenly start moving following a gradual increase of the shear stress: the way they start sliding will determine the speed and extent of the movement and, potentially, the severity of an earthquake,” explains Fabian Barras, a doctoral assistant at EPFL’s Computational Solid Mechanics Laboratory (LSMS) during this research, and first author of both articles.
Parallels between slip front and fracture
The way in which frictional sliding begins between two bodies is not as uniform as it appears. Ultrafast cameras show that slip starts at a specific point and then spreads to the rest of the surface. “This slip front dynamics is very similar to the way a crack propagates within a brittle material,” says Barras. The researchers’ first publication looks at the similarities between frictional rupture and dynamic fracture. “Although the physics of a crack and a slip front is not exactly the same, they both propagate because of a drop in the material’s load-bearing capacity behind the rupture. Using the analogy with dynamic fracture, we studied the origin of the drop of frictional stress observed in the wake of a slip front when the interface starts to move.”
The researchers then looked at the concentration of stress at the slip front and used theoretical tools from the field of rupture dynamics to study the energy balance. Unlike the situation with a crack, friction continues to dissipate energy after slip has started. During an earthquake, only part of the available energy is used to propagate the rupture front, and the remainder is dissipated by friction, mainly in the form of heat. It is here that the researchers were able to revise previously used models and achieve a better understanding of how much frictional energy is involved in the propagation of the rupture front.
They used high-performance computers to simulate seismic ruptures based on generic laws of friction, which reproduce the change in frictional force depending on the slip velocity measured between different types of materials. Using dynamic rupture theory and applying it to friction, the researchers were able to assess laboratory experiments and ensure that their predictions were correct. “We were able to validate our predictions across a wide range of experimentally observed rupture velocities. The theoretical models we developed could in the future help us better understand why certain earthquakes in nature are fast and violent, while others propagate slowly and occur over longer periods of time,” adds Barras.
Deep geothermal energy and induced seismicity
These advances in fundamental research could one day be applied to more complex models, such as those representing conditions along tectonic faults, especially where fluids are naturally present or injected into the ground. “Today, several promising technologies in the context of the energy transition – like deep geothermal energy – relies on underground fluid injection. It is important to have a better understanding of how those injections affect seismic activity. I hope to use the tools developed during my PhD to study that impact,” says Barras.
“This work shows how research developed in a civil engineering laboratory can have very interesting implications for earthquake science and lead to cutting-edge publications in areas such as physics,” says Professor Jean-François Molinari, the head of EPFL’s Computational Solid Mechanics Laboratory. Fabian Barras has also received a grant from the Swiss National Science Foundation to continue his research in a laboratory specializing in fault geology at the University of Oslo.
Caption: Between two solids in frictional contact, slip nucleates at a point on the surface (corresponding to the hypocenter of an earthquake) before spreading to the rest of the interface – just like a crack growing through a brittle material. Using numerical simulation, researchers computed the shear stress profile after the onset of slip and studied the drop of frictional stress observed behind the rupture fronts (blue area in the inset).
This research was made possible through funding from the Swiss National Science Foundation (Grant No. 162569, Fabian Barras PhD), as well as from the Rothschild Caesarea Foundation in order to kick off a collaboration between Jean-François Molinari’s lab at EPFL and Eran Bouchbinder’s theoretical physics group at Weizmann. Eran Bouchbinder would also like to thank the Israel Science Foundation for its support (Grant No. 295/16).