EPFL researchers creating the future of quantum signal amplification
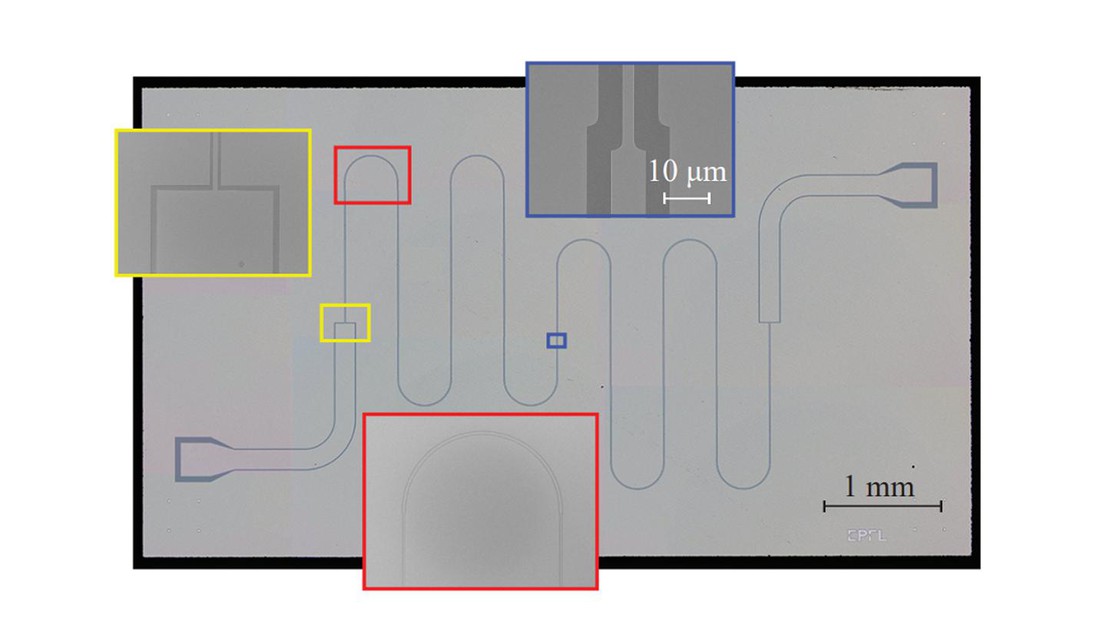
Full-size optical micrograph of the KIPA © 2024 EPFL Hybrid Quantum Circuits Laboratory
Scientists from EPFL's Hybrid Quantum Circuits Laboratory recently designed, fabricated and tested a Kinetic Inductance-based Parametric Amplifier (KIPA), a significant breakthrough in quantum signal amplification that represents an important step forward in the development of enhanced quantum sensors for technological applications and fundamental physics, potentially aiding in "tabletop precision physics".
In the field of quantum technology, extremely weak signals hold precious information about quantum phenomena, making amplification essential. In fact, in many contexts where quantum mechanics govern the behavior of modern technology, such as quantum sensing or quantum computing, the signals containing information about the quantum systems are so weak that the intrinsic noise of typical measurement electronics overwhelms them. This is where the parametric amplifier comes in.
Parametric amplification
Picture a child on a swing, effortlessly gliding back and forth. Now, imagine the child strategically moving their legs to the rhythm of their swing, subtly altering its natural frequency. By synchronizing these leg movements at precisely double the swing's frequency, the child propels themselves higher and higher—a mesmerizing display of resonance and amplification. This is the essence of parametric amplification: harnessing the power of resonance to magnify signals, much like the child elevating their swing to greater heights.
In the realm of quantum technology, parametric amplifiers play a crucial role in enhancing weak quantum signals while minimizing added noise, in accordance with the principles of quantum mechanics. This enhancement is achieved through a coherent exchange of photons between two tones of differing frequencies within a nonlinear medium. Traditionally, these amplifiers have relied on Josephson junctions, small superconducting devices with powerful nonlinear properties. However, despite their advantages, Josephson junction-based parametric amplifiers have notable limitations: they struggle with high-power signals, necessitate operation at extremely low temperatures (around 10 mK), and exhibit high sensitivity to magnetic fields, which can lead to potential malfunctions.
The presence of a magnetic field significantly affects the performance of Josephson junctions, limiting their compatibility with other quantum technologies, such as spin qubits and quantum memories, which rely heavily on external magnetic fields for operation. This sensitivity poses challenges in integrating Josephson junction amplifiers onto chips very close to the quantum device, which could otherwise reduce losses in transmission. In environments with strong magnetic fields, Josephson amplifiers stop operating effectively.
An exceptionally resilient tool
This challenge led Dr. Simone Frasca and collaborators from the Hybrid Quantum Circuits Laboratory, under the guidance of Professor Pasquale Scarlino, to implement a Kinetic Inductance-based Parametric Amplifier (KIPA), which was designed, fabricated, and tested entirely at EPFL. Their innovation utilizes the inherent nonlinearity found in “dirty” thin superconducting films, departing from previous limitations.
Published in Physical Review Applied, and also selected as an Editor’s Suggestion, their creation, the KIPA offers quantum-limited amplification with a remarkable gain-bandwidth product of 20 MHz, peak gains exceeding 40 dB, and a saturation power of -65 dBm—three orders of magnitude higher than conventional Josephson Parametric Amplifiers.
Most remarkably, the KIPA demonstrates remarkable resilience, functioning reliably even under magnetic fields of up to 6 Tesla and temperatures as high as 850 mK, a capability unprecedented in comparison to its Josephson junction-based predecessors. This magnetic field compatibility and quantum-limited noise performance position it as an invaluable tool for researchers engaged in quantum computing and sensing, particularly for applications reliant on magnetic fields, such as hole/electron-based spin qubits and spin-ensemble quantum memories. Moving forward, the team aims to expand the bandwidth of the KIPA and integrate it into a readout chain to enable fast, high-fidelity readout of spin qubits.