Bacteria under the microscope: a new growth model for tuberculosis
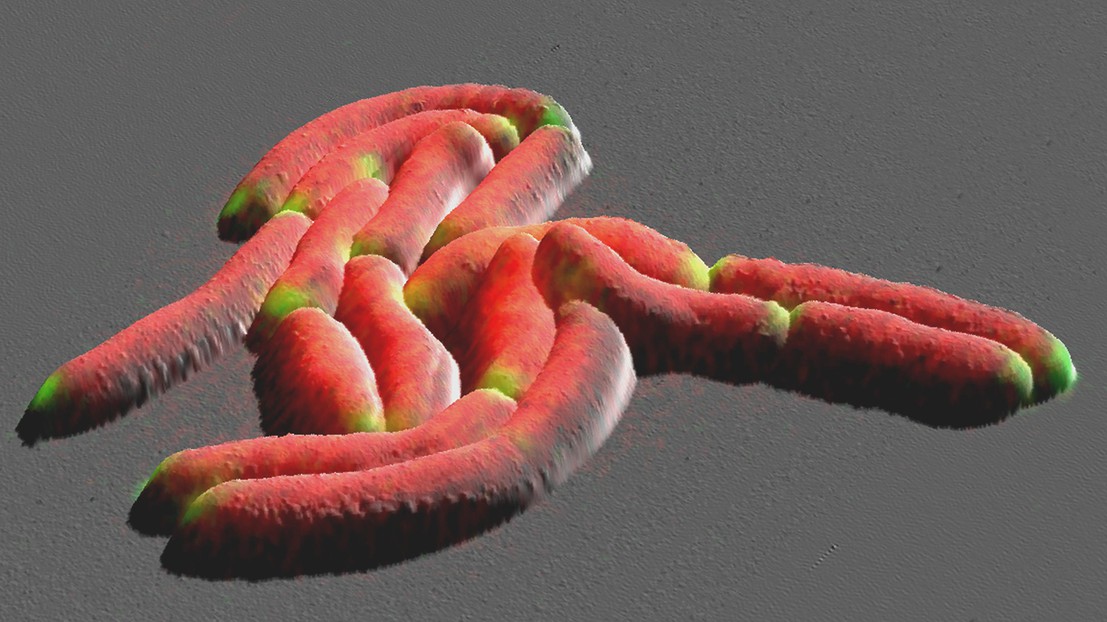
© 2020 EPFL
New research has shed light on how mycobacteria grow. This discovery could explain why some members of this family of single-celled organisms, which includes the bacillus that causes tuberculosis, can develop resistance to antibiotics
For centuries, scientists have peered down the lens of a microscope and watched as bacteria – some circular, others rod-shaped – multiply before their eyes. Yet, much about the details of how cells grow and divide is still hidden, in part because the technology to resolve this process is lacking. A team of engineers, biologists, and physicists at EPFL have now used a combination of state-of-the-art microscopes to uncover new insights into the growth of mycobacteria, a family that includes the bacillus responsible for tuberculosis. The process, described in a paper in Nature Communications, could play a part in antibiotic resistance and other bacterial defense mechanisms.
A controversy about bacterial growth
Mycobacteria are rod-shaped organisms typically measuring a few millionths of a meter in length. To multiply, they elongate then divide in the middle. Each new cell has two ends, or poles: the first inherited from the mother cell (the old cell pole) and the second formed during division (the new cell pole).
The way bacteria grow has consequences for their susceptibility to antibiotics. Until now, mycobacterial growth has been characterized by two competing theories: the unipolar model, in which growth only occurs at the old cell pole (the end inherited from the mother cell), and the bipolar model, in which both poles grow at the same rate until the cell is long enough to divide.
Physicists and engineers, led by Prof. Georg Fantner, teamed up with the laboratory of Prof. John McKinney to resolve this controversy.
Combining two imaging techniques
“The reason why these two competing models existed was that previous experiments lacked the spatiotemporal resolution to come to a definitive answer,” says Prof. Georg Fantner. To observe the growth process with high enough resolution, the team used two time-lapse imaging methods: atomic force microscopy and optical microscopy.
“With optical microscopes, we can look inside the cell, but we don’t see much detail,” says Mélanie Hannebelle, the paper’s lead author. “On the other hand, atomic force microscopes offer nanometer resolution, but can’t see inside the cell. We had to combine the two techniques to obtain the information we needed.”
A two-stage growth mechanism
The EPFL interdisciplinary team demonstrated that the truth sits somewhere between these two theories. In the beginning, the old cell pole grows at a much faster rate than the new one. But, shortly before division, the new cell pole transitions from slow to fast growth, reaching the same rate as the inherited one. The new model’s name, “biphasic,” reflects this two-stage process.
The biphasic division mechanism appears to give mycobacteria a survival advantage. Researchers in the United States recently demonstrated that, when forced to follow a more symmetrical growth pattern, the organisms become less resistant to a particular antibiotic. “We still don’t have an explanation,” says Prof. McKinney. “All we know is that there’s a link between growth mechanism and resistance; the challenge now is to find out why.” Solving this riddle is more urgent than it might sound. The best-known species of mycobacterium, M. tuberculosis, also happens to be responsible for the world’s deadliest infectious disease, which kills 1.5 million people every year – and drug-resistant strains are becoming increasingly common.
A biphasic growth model for cell pole elongation in mycobacteria. Mélanie T. M. Hannebelle, Joëlle X. Y. Ven, Chiara Toniolo, Haig A. Eskandarian, Gaëlle Vuaridel-Thurre, John D. McKinney & Georg E. Fantner. Nature Communications volume 11, Article number: 452 (2020)