A novel on-chip microwave-optical transducer
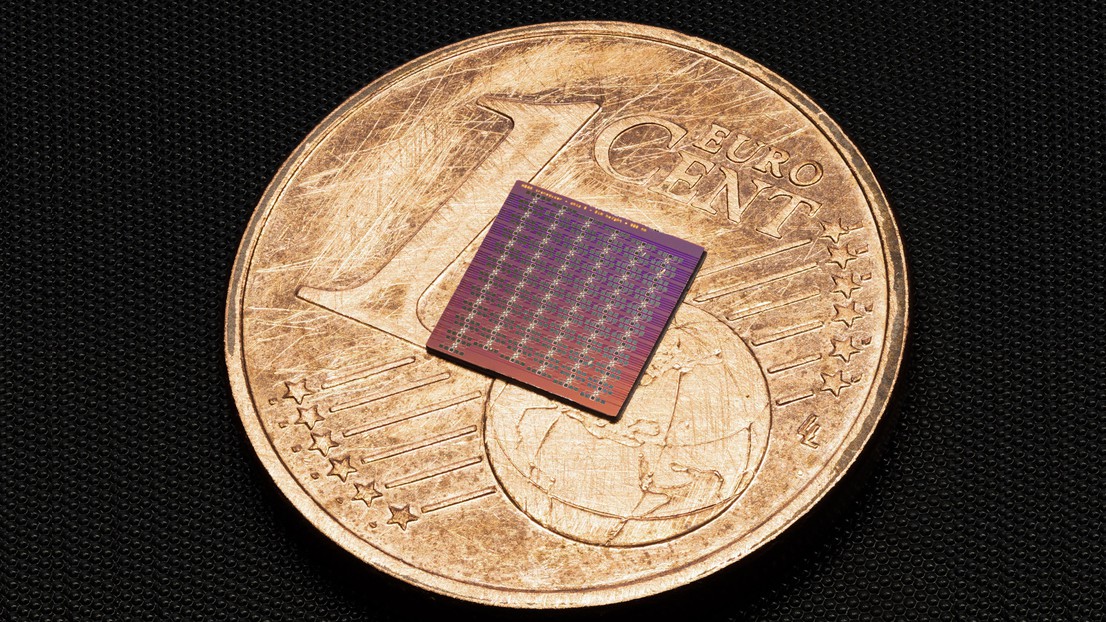
© 2024 EPFL : Dark-field optical micrograph of the microwave-optical transducer, including the photonic circuitry and piezoelectric actuators, shown in bird's-eye view.
EPFL and Purdue scientists create a coherent interface between microwaves and near-infrared light to help scale up future quantum networks.
A team of researchers at EPFL, led by Prof. Tobias Kippenberg, in collaboration with Prof. Sunil Bhave's group at Purdue University, has achieved a key step in interfacing microwave superconducting circuits with optical photons, which could overcome critical challenges in scaling up these systems for next-generation computing.
Superconducting circuits, favored for their high nonlinearity due by quantum mechanical tunneling of paired electrons across Josephson junctions, need to operate at only fractions above absolute-zero temperatures within dilution refrigerators. Scaling up these systems up is challenging as it requires adding more coaxial lines, low-noise amplifiers, and attenuators, which introduces excessive heat and strains the cooling capacity of the fridges.
To overcome this problem, the researchers explored the use of optical fibers—the backbone of modern global communications networks. They generate minimal heat within dilution fridges, and are most effective at transmitting light at near-infrared wavelengths. This requires a coherent interface between the microwaves from the superconducting circuits and the optical frequencies. Optical photons, which are robust to thermal noise due to their high frequencies, facilitate long-distance signal transmission between distant fridges to form extensive networks.
Conventional integrated coherent interfaces, typically made of nanoscale suspended beam structures or anisotropic crystals patterned into photonic waveguides, suffer from poor power handling and narrow bandwidth. The EPFL team overcame these limitations by fabricating piezoelectric actuators on photonic waveguides made from high-quality silicon nitride, which drives bulk acoustic resonances in the acoustic cavity formed by the surrounding silicon oxide cladding. This design improves thermalization, allows stronger optical pumping fields, and provides multiple conversion channels with large bandwidth due to the acoustic cavity overtones. Moreover, the fabrication processes are compatible with CMOS foundry offerings, ensuring the scalability and integration of this technology with existing semiconductor manufacturing infrastructure. A chip smaller than a five-cent Swiss Franc coin can house 65 such devices, thanks to their extremely compact footprint.
The researchers have demonstrated bidirectional microwave-optical frequency conversion. Their system can operate using both Stokes and anti-Stokes scattering, paving the way for noiseless frequency conversion and potential entanglement between microwave and optical photons.
A major advantage of this new approach is the chip’s ability to generate significantly more microwave power than other transduction methods, with a bandwidth exceeding current state-of-the-art technologies. These down-converted drives are powerful enough to control superconducting qubits or read their state. Additionally, the photonic waveguide's cladding supports multiple acoustic modes, allowing a single transducer to connect with several superconducting qubits, reducing the need for multiple microwave wires and amplifiers.
The work has now been published in Nature Communications. The team is now focusing on further improving the efficiency of the transducer. Higher efficiencies would enable faster operations, allowing more computations to be performed within the qubits' coherence time. The resulting faster generation of entangled microwave-optical photon pairs could be used to teleport information between remote qubits—a promising method for long-range two-qubit interactions.
Air Force Office of Scientific Research (AFOSR)
Swiss National Science Foundation (SNSF)
European Research Council (ERC)
EU H2020 research and innovation program
United States Air Force Research Laboratory (AFRL)
United States National Science Foundation (NSF)
European Space Agency (ESA)
T. Blésin, W. Kao, A. Siddharth, R. N. Wang, A. Attanasio, H. Tian, S. A. Bhave, and T. J. Kippenberg, Bidirectional Microwave-Optical Transduction Based on Integration of High-Overtone Bulk Acoustic Resonators and Photonic Circuits, Nat Commun 15, 6096 (2024).