A faster path to optical circuits
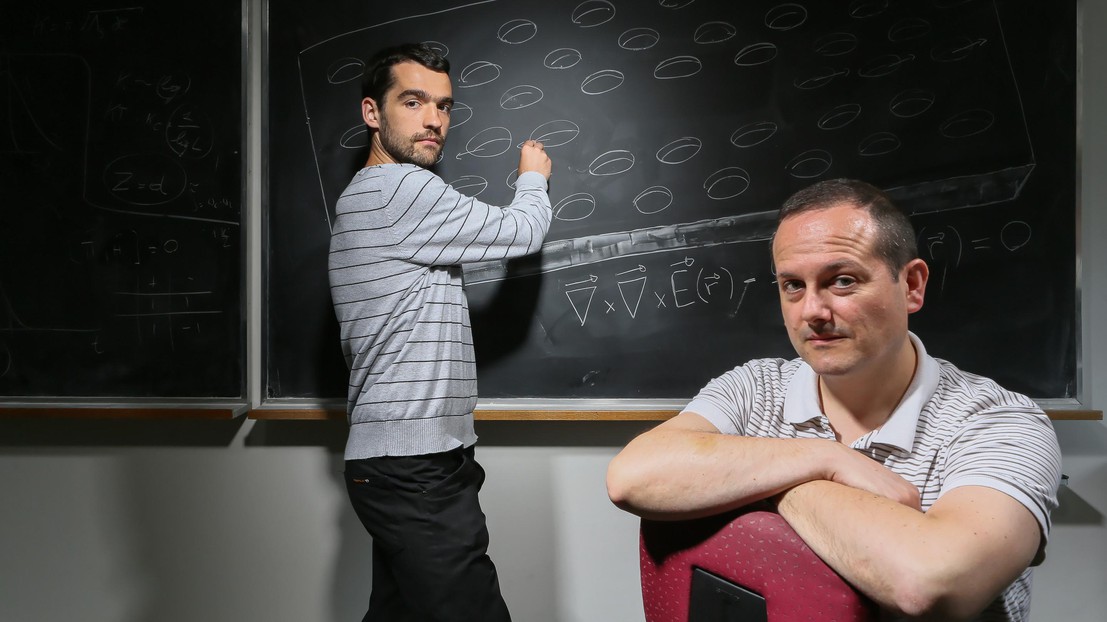
Minkov (L) and Savona (R) with a PCN design ©Alain Herzog/EPFL
Scientists at EPFL develop a fast and effective method for optimizing photonic crystal nanocavities. The method has led to the design of new-generation structures that may advance the future of optical circuits.
Just as electronic circuits work with electrical charges, optical circuits process pulses of light, which gives them a distinct advantage in terms of speed. Optical technologies are therefore the object of intense research, aiming to develop novel optical devices that can control the flow of light at the nanometer scale. EPFL scientists have developed a new method that can optimally design a widely-used class of optical devices with unprecedented effectiveness. Their designs have been fabricated in the US, at the University of Rochester, and successfully tested in Italy, at the University of Pavia. In two publications in Applied Physics Letters and Scientific Reports, the result of this collaboration will considerably speed up the development of optical circuits.
In order to use light for encoding information in future communication systems, it is first necessary to regulate its flow and retain it for even a fraction of a second, to avoid signal “traffic jams”. This is achieved by optical nanocavities, which are arrangements of mirrors that force light to bounce between them and can therefore retain it in a small space. They are widely used in lasers and optical devices, and are made from a variety of materials, such as silicon. Optical nanocavities are also ideal for building optical circuits, which regulate the flow of light just like conventional transistors regulate the flow of electrons. Optical circuits can also be integrated with electronic circuits into extremely compact structures to increase performance in information and communication technologies.
The most promising optical nanocavities are built inside structures called “photonic crystals”, giving them the name “photonic crystal nanocavities (PCNs)”. PCNs operate like the components of an electronic circuit, except they control the flow of light instead of the flow of electrons. Because of their complex geometry, the optimization of PCNs is a challenging task, requiring lengthy computer simulations of hundreds of possible designs before selecting the best for nanofabrication.
The group of Vincenzo Savona at EPFL has developed a novel method to design, simulate and optimize PCNs and applied it to one of the most common PCN types, used widely in commercial optical circuits. The goal was to maximize the PCN quality factor – a term that refers to the length of time the nanocavity can hold a photon before it escapes. “Ideally you want to confine light as long as possible and inside a volume as small as possible”, says Savona. “The nanocavities we are optimizing are smaller than the optical wavelength itself (about 1 micrometer) and have a quality factor higher than 1 million, meaning that a photon can go back and forth inside the nanocavity more than 1 million times before escaping.”
The scientists perfected a computer modeling algorithm that can simulate a single PCN structure in matter of minutes –much faster than the several hours typically required by commercial tools. As PCN optimization requires simulating thousands of different structures, this new approach offers considerable advantages in terms of time and effectiveness.
An 'evolutionary' process
The fast algorithm was combined with an optimization software tool that is referred to as ‘genetic’ or ‘evolutionary’ because it uses a kind of ‘natural’ selection to choose the best nanocavity structures. The “evolutionary” process begins by regarding each PCN structure as an individual in a population, like animals in a herd. The individuals then “breed” between them, meaning that two single PCN structures combine to create a new one that is a cross-over between its two parents.
As PCN generations succeeded one another, Savona’s algorithm rapidly simulated the structures, and the evolutionary software selected the best individuals – in this case, the ones that showed the best “fitness” with respect to a desired quality, e.g. photon lifetime or frequency. The entire process is fully automated and typically involves a few hundreds of generations, each including one hundred individuals. “Our fast method means that an optimization will typically take a couple of days on a computer”, says Savona.
The best PCN designs were sent to a laboratory in the US to be nanofabricated on a silicon platform. From there, the PCNs were shipped to another laboratory in Italy, where they were successfully tested. “In the end, we improved all of the most widespread PCN designs by a factor of 10-20 in terms of lifetime of the confined photons”, Savona explains. The devices show some of the highest quality factors ever measured in PCNs, which can have a tremendous impact on the development of integrated photonic circuits.
---
This work is part of a larger study involving the EPFL Laboratory of Theoretical Physics of Nanosystems, Professor Romuald Houdré’s lab at EPFL’s Laboratory of Quantum Optoelectronics, the University of Rochester Department of Physics and Astronomy, and the University of Pavia Department of Physics. The original idea and its theoretical demonstration have been simultaneously published in Scientific Reports, authored by Vincenzo Savona and his PhD student, Momchil Minkov. The Applied Physics Letters paper shows its first practical application in a device.
References
Lai Y, Pirotta S, Urbinati G, Gerace D, Minkov M, Savona V, Badolato A, Galli M. Genetically designed L3 photonic crystal nanocavities with measured quality factor exceeding one million. Applied Physics Letters 104, 241101 (2014); DOI: 10.1063/1.4882860
Minkov M, Savona V. Automated optimization of photonic crystal slab cavities. Scientific Reports 4, 5124; DOI: 10.1038/srep05124