Uncovering the inner workings of hydrogen-processing enzymes
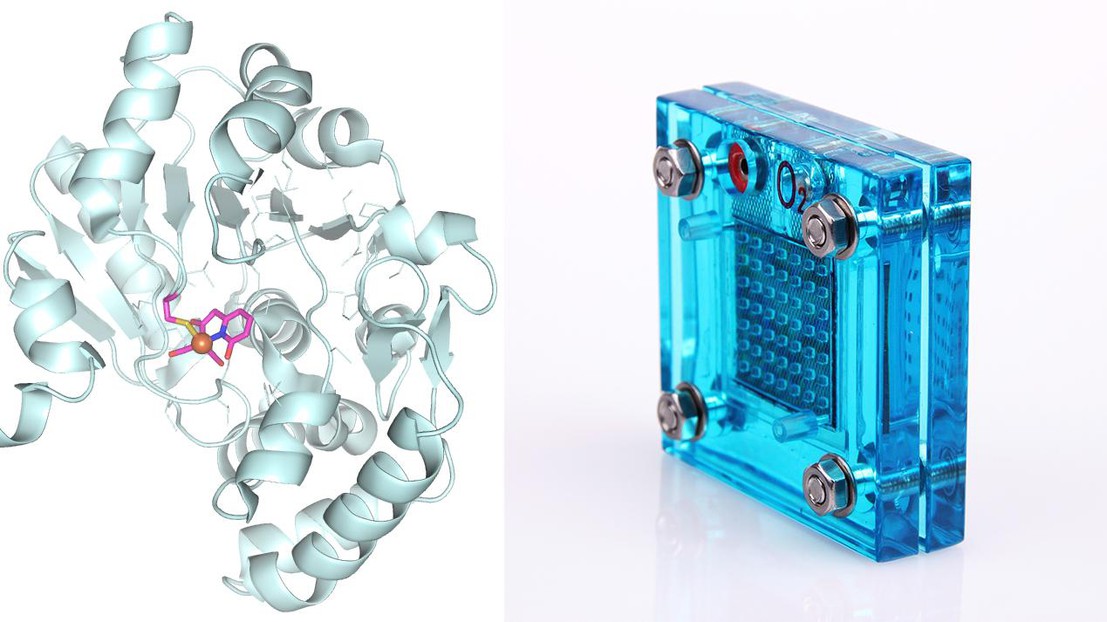
Hydrogenase and a fuel cell © Xile Hu (EPFL)/Thinkstock
EPFL scientists have determined the mechanism of a hydrogenase enzyme that can be used for hydrogen processing. The work can potentially improve future hydrogen-energy technologies like fuel cells and hydrogenation plants.
As the world moves progressively towards renewable energy sources, e.g., being able to store such energy is developing into a challenge itself. Storing energy in the form of hydrogen is a promising solution. Scientists are designing systems that will mimic enzymes called hydrogenases, which process hydrogen in their energy metabolism. However, the way hydrogenases work is not very well understood. Scientists at EPFL and the Max Plank Institute have used synthetic molecules to decipher which parts of a hydrogenase are key to the reaction. The work, which may greatly improve hydrogen processing, is published in Nature Chemistry.
Hydrogenases are some of the oldest enzymes on Earth, producing hydrogen fuel for various bacteria species. There are different families of hydrogenases, each using different metal ions to bind and catalyze their hydrogen-processing reactions reversibly. The forward reaction begin by cleaving hydrogen into a proton and a hydride ion (H-), which is followed by oxidation of hydride as in fuel cells, or hydrogenation of biological substrates as in industrial plants. The reverse reaction produces hydrogen gas (H2), which can be stored away for future energy use.
The lab of Xile Hu at EPFL, working with the lab of Seigo Shima at the Max Plank Institute for Terrestrial Microbiology, as well as colleagues in Germany and China, have now solved the mystery by using cofactor mimics of a hydrogenase. Cofactors are molecules that help enzymes perform their function; without its cofactor, hydrogenase remains inactive.
The researchers focused on [Fe]-hydrogenase, which contains a single iron atom in its cofactor. The cofactor (referred to as “FeGP” for “iron-guanylylpyridinol”) is located at the enzyme’s active site, where the hydrogen-cleaving reaction takes place.
“We know a lot about the structure and the properties of [Fe]-hydrogenase, but very little as to how it activates hydrogen in the first step of the reaction,” says Xile Hu. It is also known that the [Fe]-hydrogenase apoenzyme (enzyme without its cofactor) can be reactivated by reconstituting it with its isolated cofactor. In this study, the scientists built two synthetic FeGP cofactor mimics: one with a 2-hydroxy pyridine group and one with a 2-methoxy-pyridine complex. They then reconstituted the [Fe]-hydrogenase enzyme with the synthetic small molecules.
“The advantage here is that the cofactor mimics can be systematically modified and are easier than the enzyme for characterization,” explains Xile Hu. “However, neither mimic can bind or activate hydrogen by itself.”
The results showed that the cofactor with hydroxyl-pyridine restored the activity of the [Fe]-hydrogenase, while the cofactor with methoxy-pyridine left it inactive. Wanting to get a full picture of what makes the enzyme work, the team followed up with advanced “density functional theory” calculations, which are a quantum mechanical approach used to model the chemistry of complex systems.
The work provided unique insights into the mechanism by which [Fe]-hydrogenase cleaves hydrogen in the first step of the reaction. Specifically, it appears that the 2-hydroxy group of the cofactor is deprotonated and then acts as an internal base for cleavage of hydrogen.
Understanding how hydrogenases work can help scientists design more efficient systems for hydrogen production and activation. “Energy technologies in the future will rely upon the development of new catalysts for activating hydrogen,” says Xile Hu. “So we need to develop robust, efficient, and cost-effective catalyst systems – and this work provides a major guideline towards that.”
This work represents a collaboration of EPFL’s Laboratory of Inorganic Synthesis and Catalysis with the Max Plank Institute for Terrestrial Microbiology. It also involved EPFL’s Laboratory for Computational Molecular Design, the Japan Science and Technology Agency (JST), the Harbin Institute of Technology, and the Freie Universität Berlin. The study was funded supported by the Max Planck Society, the JST, the National Natural Science Foundation of China, and the Swiss National Science Foundation.
Reference
Shima S, Chen D, Xu T, Wodrich MD, Fujishiro T, Schultz KM, Kahnt J, Ataka K, Hu X. Reconstitution of [Fe]-hydrogenase using model complexes.Nature Chemistry 02 November 2015. DOI: 10.1038/nchem.2382